The basic principle of all li-ion batteries is: li-ions on the run.
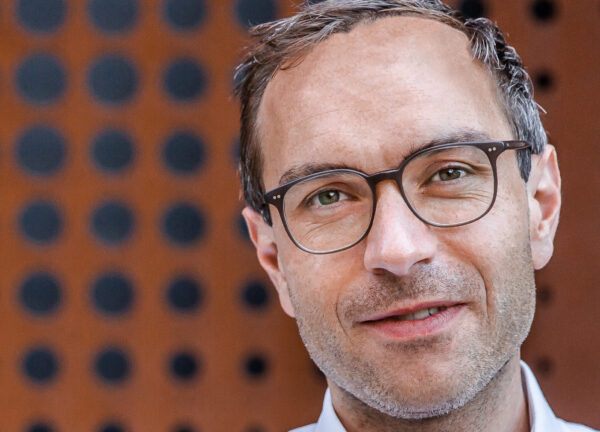
Image: volytica diagnostics GmbH
In a fully charged battery cell, billions of lithium (Li) atoms are trapped in the “anode” porous reservoir, typically a thin sheet of a particular material. The anode and the opposite side, the “cathode,” are conductively connected to the positive and negative terminals of the battery cell. Hundreds or thousands of cells are assembled into a battery system. In the anode, lithium atoms, or ions, are ready to travel to the opposite reservoir, the cathode.
This cumbersome parkour is triggered by externally connecting the anode and cathode to the rest of the asset, for example inverters, motors, and power sources. Once the circuit is closed, the ions queue to exit the anode for the cathode, each releasing an electron which travels a long tour, via the external circuit, to power the asset – the stream of electrons leaving batteries is the electric current. Each ion and electron will reunite on the other side until the battery is charged again.
Released ions force their way through an intermediate medium designed for swift transport while keeping anode and cathode separate – a distance of only micrometers – to avoid short circuiting, with catastrophic results. This intermediate material must be permeable, light, and thin, but insulating and robust, causing headaches for engineers and reducing battery capacity per volume and weight. Low-viscosity chemical liquids or polymers – the electrolyte – are submerged in a matrix, or “separator” in the current mainstream approach. Another option is solid-state li-ion batteries, with liquid electrolyte and separator replaced by a solid material, often exotic ceramics. The topic of solid-state batteries, however, is an article all on its own.
After passing through electrolyte and separator, lithium ions slow to avoid crowding upon entry to the cathode and, one-by-one, migrate slowly into their new host material, finding energetically advantageous positions in the cathode lattice. Think of passengers boarding an empty train: the first find numerous free seats and rapidly occupy them, making space for queueing followers. The more seats that are taken, however, the slower the boarding process becomes. Macroscopically speaking, battery charging is quick initially but the longer you charge, the slower it gets. In electric cars, the final 20% of charge can take as long as the initial 80%.
Degradation
The whole process is somewhat more complex than boarding a train, however. It involves ion interaction with a multitude of different materials, at different speeds, temperatures, stress levels, and in different processes. Battery degradation issues hinge on factors such as the effect of temperature on electrolyte viscosity, and thus the process speed of this crucial voyage. Also, as the “best seats” are taken, step by step, the host materials slightly expand, adding mechanical expansion stress and potentially leading to internal cracks and fissures. It is obvious that the way of charging, discharging, and operating a battery will dramatically affect the magnitude and rate of degradation processes that lead to the well-known loss of capacity, and also to safety-critical side processes.
In terms of different battery chemistries and the abbreviations mentioned above, the anode reservoir is traditionally made of graphite, albeit with a myriad of secret additives, manufacturing, and processing variations. LTO batteries are an exception which will be considered shortly.
For cathodes, things become more complicated. Cathode materials made of varying amounts of nickel (Ni), manganese (Mn), controversial cobalt (Co) – and sometimes also aluminum (Al) – plus other secret additives, are referred to as NMC or NCA (nickel-cobalt-aluminum) devices. Such cathodes typically have high energy density, meaning they can accommodate a high number of lithium ions per volume but have shorter lifetimes, lower safety margins, and a bigger price tag than rival chemistries as a result.
To satisfy the automotive industry’s hunger for energy density and range, engineers crank up the nickel content – the first NMC batteries had equal parts of the three components, were dubbed NMC 1-1-1, and offered decent energy density and robust safety characteristics. Nowadays, industry adds eight times more Ni than manganese and cobalt, for NMC 811 batteries. These offer much greater range but significantly shorter lifetime and safety characteristics. That’s right, average li-ion NMC lifetimes and safety are decreasing.
By contrast, the second dominant cathode type is lithium iron-phosphate, also known as LiFePO4 or lithium ferro-phosphate. These cathodes feature lower energy density than NMC devices but are more robust and – at least traditionally – more affordable. Increasingly popular, LFP-based batteries are almost entirely manufactured by Chinese producers. LFP systems are handicapped by the fact their voltage varies little over a wide range of battery state-of-charge levels. State of charge, or SOC, is the amount of charge, or energy, in a battery. Less flexible voltage makes balancing and control of systems, as well as determining SOC, difficult and renders LFP applications generally prone to unexpected operational problems and downtime, further aggravating their lower energy density.
LTO devices
As mentioned, there is an exotic battery variant which uses lithium-titanate (lithium titan oxide, or LTO) for the anode, rather than graphite, sometimes paired with an LFP cathode. These devices offer very low energy density (even lower than legacy nickel-metal hydride, NiMH, chemistry) and can cost 50% to 150% as much as NMC cells because they offer between 10 times and 30 times better safety and longevity performance than LFP and NMC systems.
These are broad brush strokes, however, and the terrific variety of li-ion chemistries available offers cell manufacturers the opportunity to push performance in one direction or another. While characteristics can vary as a result, though, a giant leap in energy density for li-ion, without negative effects on aspects such as lifetime, cannot realistically be expected in the near future.
Volytica diagnostics will be holding a pv magazine webinar on batteries and their deployment in the energy transition, at 4 p.m. CEST on Wednesday, Sep. 20.
About the author: Claudius Jehle is founder and CEO of Volytica diagnostics GmbH, a company focusing on easy, safe, and independent battery quality and health assessment. Claudius studied mechanical engineering and system identification at TU Munich and TU Delft and led the energy storage diagnostics and telematics research group at the Fraunhofer Institute for Transportation and Infrastructure Systems IVI, in Dresden, until the spin-out of Volytica in 2019.
The views and opinions expressed in this article are the author’s own, and do not necessarily reflect those held by pv magazine.
This content is protected by copyright and may not be reused. If you want to cooperate with us and would like to reuse some of our content, please contact: editors@pv-magazine.com.
1 comment
By submitting this form you agree to pv magazine using your data for the purposes of publishing your comment.
Your personal data will only be disclosed or otherwise transmitted to third parties for the purposes of spam filtering or if this is necessary for technical maintenance of the website. Any other transfer to third parties will not take place unless this is justified on the basis of applicable data protection regulations or if pv magazine is legally obliged to do so.
You may revoke this consent at any time with effect for the future, in which case your personal data will be deleted immediately. Otherwise, your data will be deleted if pv magazine has processed your request or the purpose of data storage is fulfilled.
Further information on data privacy can be found in our Data Protection Policy.