An introduction to both sides of this issue, and prospects for responsible compromise, were presented in the November 2019 issue of pv magazine.1 In the following article, we highlight our related study of the life-cycle lead emissions and toxicity potential of LHP-PVs in comparison to several US grid electricity mixes, published in January 2019 in Energy,2.
We found that the lead emissions and toxicity potential associated with LHP-PVs are less than those associated with grid electricity, but we caution that proper industrial hygiene during manufacturing, robust encapsulation for durable operation, and end-of-life management are critical for responsible deployment of this technology.
Lead in solar
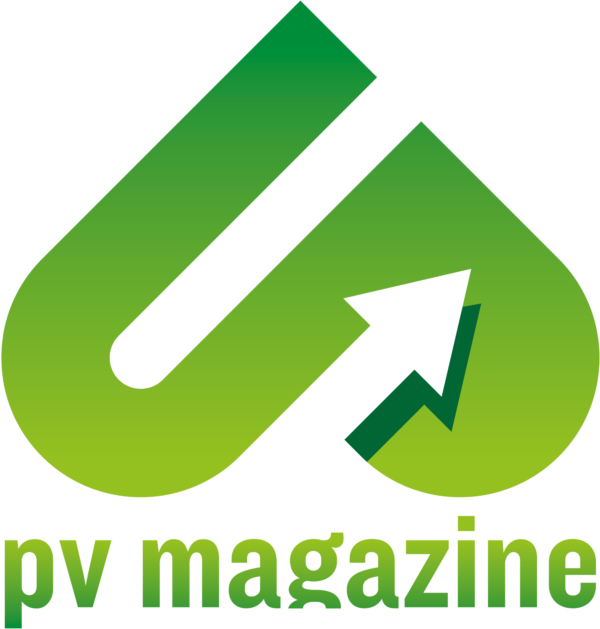
PV does not emit anything during operation, but, over its life-cycle, emissions are generated from the use of fossil-fuels in the extraction and production of materials, and in the manufacturing and installation of PV components and systems. Nonetheless, these emissions are always very small compared to those from conventional combustion-based generators of electricity. The case for CO2 emissions has been well documented: over their lifetime, they range from 10 g CO2-eq / kWh for CdTe PV systems installed in high-irradiation (2300 kWh/(m2-yr) locations, to 80 g CO2-eq / kWh for Chinese single-crystalline silicon PV systems installed in low-irradiation (1000 kWh/(m2-yr) locations,3 compared to ~1000 g CO2-eq/kWh for electricity from coal. However, in the case of the rapidly emerging LHP-PV technology, concerns are raised regarding the potential of emissions of lead from the life-cycles of LHP-PV, including at their end-of-life. This warranted an evaluation of lead emissions from PV and comparisons with emissions in other power generation life-cycles.
LHP-PVs have rapidly increased in efficiency, exceeding 25% this year for small-scale devices and surpassing the champion lab-scale efficiencies of other thin film technologies developed over decades.4 While they were initially plagued by extremely poor stability, advances in both materials chemistry and encapsulation have led to improved performance in accelerated aging tests, portending operational lifetimes that may reach the multi-decade range. These achievements have attracted significant investment into LHP-PVs, which are now in early stages of commercialization- both as single junction devices and as perovskite-perovskite and perovskite-silicon two-terminal tandems. Despite this great excitement, concerns remain, because of the presence of toxic lead in the absorber layer.
Lead is a critical element in the LHP absorber, which exhibits the perovskite crystal structure with formula ABX3, where A is a large cation such as organic methylammonium or inorganic cesium, B is lead, and X is a halogen such as iodine. Efficiencies of lead-free perovskite PV remain below 12% so far, with the best performance using tin. It is still unclear whether a new candidate composition will emerge with additional research or the optoelectronic properties endowed by lead are inherently superior. If lead is indeed a pre-requisite for high efficiencies, questions remain regarding whether benefits of enhanced deployment of PV may outweigh the risks of deploying lead-containing devices.
Life cycle assessment reveals the benefits from offsetting conventional electricity
For a holistic and comparative evaluation of benefits and risks of LHP-PV, a life-cycle framework is necessary. Our initial study on the topic was published earlier this year in Energy.2 We compared lead emissions and toxicity potential of LHP-PVs to three different representative U.S. grid mixes, including case studies where proper disposal resulted in no release of lead into the environment; we also considered a worst-case scenario where all lead from the panels was accidentally released to groundwater.
The amount of lead in LHP-PVs is actually quite small – only 1.4 g/m2 for a 1-micron thick absorber layer. Even this estimation is conservative; record-efficiency small-area cells often used absorbers four times thinner, and embodied lead would be correspondingly less. Considering large-scale lead supply, the entire electricity demand of the United States could be met with 2,400 GWp of LHP-PV, which corresponds to only 17,000 metric tons of embodied lead for a micron-thick absorber. By comparison, 1.6 million metric tons of lead are used annually in the United States, primarily for lead acid batteries.
We have tracked the lead emissions associated with different categories of LHP-PV manufacturing and operation, and found that the majority would be from the Pb content in the PV balance of systems (power electronics, cables and mounting structures), which are common to all PV types. Due to stringent Pb emission thresholds (by OSHA, EPA and state agencies) and the very small amounts of Pb in the panels, emissions from Pb mining and smelting operations are negligible, and emissions in LHP-PV panel manufacturing should be very small. Our preliminary examination showed that life-cycle lead emissions were ~2 g/m2 during normal operation and 3.4 g/m2 in the case of accidental releases (Figure 1a).
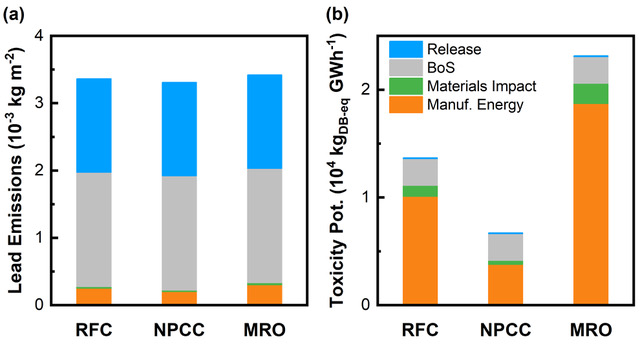
Furthermore, accidental release of lead during operation seems to have a negligible effect on the toxicity potential of LHP-PV life-cycles. Instead, the dominant contributor to toxicity potential was toxic gas emissions from the production of energy required for manufacturing and upstream stages (Figure 1b). Different grid mixes result in significantly different toxicity potential depending on the relative composition of fossil fuels, nuclear, and renewables. Using “clean” electricity to manufacture PV, therefore, dramatically reduces their environmental impact.
Despite the presence of lead in LHP-PV, these simple comparisons show that the emissions of Pb and the toxicity potential during their entire life-cycles are lower than those from conventional coal-intensive electricity grids, as the later routinely emit lead and other toxic heavy metals from fuel combustion.
While combustion emissions control technology has improved dramatically, small and continuous emissions of volatile heavy metals persist during operation.5,6 Just as economic and energy payback times are often discussed for PVs, payback times for lead emissions and toxicity potential can also be calculated. Such payback times depend on the grid mix used to produce PV components and systems, and the grid mix displaced by their deployment, as shown in Figure 2. For the considered grid mixes, lead emissions payback times were in the range of four to eight years with proper disposal and eight to 14 years when catastrophic release was assumed. Payback times for aggregated toxicity potential, which also includes other heavy metals, are under six years for all of the grid combinations considered. Presumably LHP-PVs will not be commercialized without operational lifetimes of at least 15-20 years, rendering them an environmentally favorable option compared to conventional electricity.
Our studies assumed 17% efficiency and 184 kWh/m2 of embodied energy7 of module manufacturing, but these conclusions are robust over reasonable values for these parameters.
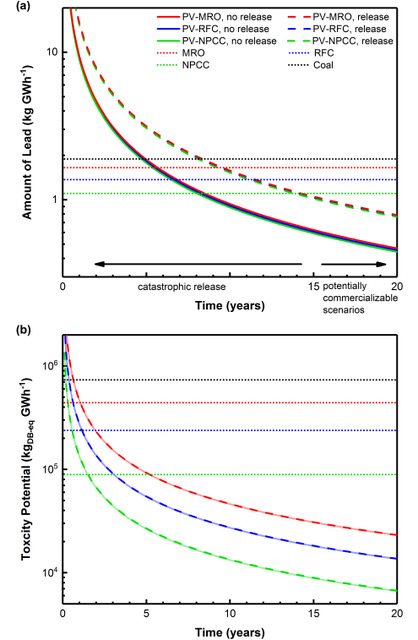
Managing risks during manufacturing, deployment, and end-of-life
While our study illustrates the positive outcomes of LHP-PVs from a general perspective, care must be taken to minimize exposure risks, both during manufacturing and during deployment. In manufacturing settings, workers must be protected against accidental exposure to lead compounds through inhalation, skin contact, or ingestion. Engineering controls and good industrial hygiene have prevented any detectable uptake of cadmium by workers in CdTe manufacturing.8 Similar care will be needed with LHP-PVs.
Durable glass-glass modules with proper encapsulation are critical to minimize the risk of lead leaching into the environment. Such protection is necessary, because lead halide perovskites decompose upon exposure to water, and the lead-containing component that remains, PbI2, is highly soluble (solubility constant on the order of 10-8, which is much higher than CdTe at 10-27). The worst-case scenario of panels cracking, leaching of lead from the now-exposed perovskite layer, and human uptake of undiluted runoff water is highly unlikely. The risk of using lead in solar cells is therefore different from risks associated with lead in plumbing or leaded gasoline, in which human uptake of small amounts of lead was unavoidable.
Deploying perovskite PVs in solar farms owned by businesses or utilities, rather than in many small rooftop arrays would help to ensure proper disposal at the end of the operational lifetime of the system. Of course, a mandatory PV recycling scheme, which is important for sustainable large growth of PV, will greatly improve this picture.9 Early efforts to consider end-of-life management are already underway, but more work will be needed if significant amounts of LHP-PV are deployed.10
Conclusions
In conclusion, under a reasonable set of assumptions, displacing electricity mixes typical of U.S. grids with lead halide perovskite PV could actually reduce lead emissions by a factor of two to four over a 20-year lifetime. Toxicity potential of perovskite PVs could be up to 20 times lower than that of current grid electricity. Because the embodied energy contributes the largest fraction of their life-cycle toxicity potential, future research efforts should focus on energy-efficient manufacturing strategies and on increasing the operational lifetime of these devices. We again emphasize that our work is preliminary, as it employed generalized assumptions regarding global-scale material and energy flows; and we stress the importance of follow-up studies of occupational and local population risk assessments as well as proper end-of-life management of lead-containing PV systems.
References
- O’Donnell, “A Responsible Compromise,” pv magazine, p. 64, Nov. 2019.
- Billen, E. Leccisi, S. Dastidar, S. Li, L. Lobaton, S. Spatari, A.T. Fafarman, V.M. Fthenakis, and J.B. Baxter, “Comparative Evaluation of Lead Emissions and Toxicity Potential in the Life Cycle of Lead Halide Perovskite Photovoltaics,” Energy, 166C, 1089 (2019).
- Leccisi, M. Raugei, and V. Fthenakis, “The energy and environmental performance of ground-mounted photovoltaic systems—a timely update,” Energies, 9, 622 (2016).
- National Renewable Energy Laboratory, Best Research-Cell Efficiencies, https://www.nrel.gov/pv/assets/pdfs/best-research-cell-efficiencies.20190923.pdf
- Fthenakis, “Life Cycle Impact Analysis of Cadmium in CdTe Photovoltaic Production,” Renewable and Sustainable Energy Reviews, 8, 303 (2004).
- Siler-Evans, I.L. Azevedo, and M.G. Morgan, “Marginal Emissions Factors for the U.S. Electricity System,” Environmental Science & Technology, 46, 4742 (2012).
- Celik, Z.N. Song, A.J. Cimaroli, Y.F. Yan, M.J. Heben, and D. Apul, “Life Cycle Assessment (LCA) of perovskite PV cells projected from lab to fab,” Solar Energy Materials & Solar Cells, 156, 157 (2016).
- Rix, J.D.T. Steyl, J. Rudman, U. Terblanche, J.L. van Niekerk, “First Solar's CdTe module technology performance, life cycle, health and safety impact assessment,” CRSES 2015/06 (2015).
- Fthenakis, “Considering the total cost of electricity from sunlight and the alternatives,” Proceedings of the IEEE, 103, 283 (2015).
- M. Kadro and A. Hagfeldt, “The end-of-life of perovskite PV,” Joule, 1, 29 (2017).
About the authors
Jason B. Baxter a, Pieter Billen b, Enrica Leccisi c, Sherif A. Khalifa a, Sabrina Spatari d, e, Aaron T. Fafarman a, and Vasilis Fthenakis c
- a Drexel University, Department of Chemical and Biological Engineering, 3141 Chestnut Street, Philadelphia, Pennsylvania 19104, United States
- b University of Antwerp, BioGEM, Salesianenlaan 90, 2660 Hoboken, Belgium
- c Columbia University, Center for Life Cycle Analysis, 500 W 120th Street, New York, NY 10027, United States
- d Drexel University, Department of Civil, Architectural and Environmental Engineering, 3141 Chestnut Street, Philadelphia, Pennsylvania 19104, United States
- e Technion – Israel Institute of Technology, Faculty of Civil and Environmental Engineering, Haifa 3200002, Israel
The views and opinions expressed in this article are the author’s own, and do not necessarily reflect those held by pv magazine.
This content is protected by copyright and may not be reused. If you want to cooperate with us and would like to reuse some of our content, please contact: editors@pv-magazine.com.
2 comments
By submitting this form you agree to pv magazine using your data for the purposes of publishing your comment.
Your personal data will only be disclosed or otherwise transmitted to third parties for the purposes of spam filtering or if this is necessary for technical maintenance of the website. Any other transfer to third parties will not take place unless this is justified on the basis of applicable data protection regulations or if pv magazine is legally obliged to do so.
You may revoke this consent at any time with effect for the future, in which case your personal data will be deleted immediately. Otherwise, your data will be deleted if pv magazine has processed your request or the purpose of data storage is fulfilled.
Further information on data privacy can be found in our Data Protection Policy.